Witnessing a Quantum Avalanche
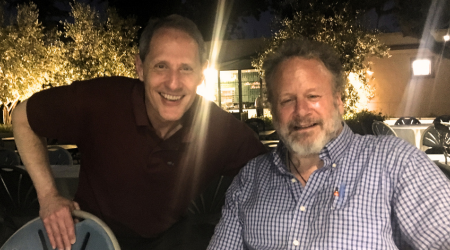
Witnessing a Quantum Avalanche
This article is a summary of "Quantum Barkhausen noise induced by domain wall cotunneling", by C. Simon, D.M. Silevitch, P.C.E. Stamp, and T.F. Rosenbaum., published in PNAS vol. 121 no.13, March 26, 2024.
In a ground-breaking discovery, scientists have observed a literal quantum avalanche! PHAS faculty Philip Stamp, Director of the Pacific Institute of Theoretical Physics (PITP), along with Thomas F. Rosenbaum, President of the California Institute of Technology and colleagues explain their findings in "Quantum Barkhausen noise induced by domain wall cotunneling," published in Physics World and PNAS.
The world is immersed in constant 'noise' – a host of random ambient disturbances from the ground beneath us, the depths of oceans, the atmosphere, and even the expanse of space. This noise, whether natural or human-induced, records the ceaseless movement and activity occurring at every scale, from the macroscopic to the microscopic.
Barkhausen Noise
Barkhausen Noise, a phenomenon known for over a century in classical magnetic systems, arises from the thermal motion of the magnetic domain walls which separate magnetic domains. Barkhausen detected this noise by wrapping a coil around a magnet, and connecting this to a loudspeaker – the audible noise captures the random motion of the domain walls. Occasionally, however, there is a loud burst of noise, when many walls move cooperatively – this is an “avalanche” process.
Whether it's the subtle shifting of magnetic domains, the rumble of shifting earth triggering a landslide, or the interplay of melting and freezing ice particles setting off a snow avalanche, these occasional “avalanche” surges of activity are observed across a huge variety of systems. Other examples include seismic events, photomultiplier tubes, lightning bolts, forest fires and epidemics, and, on longer time scales, to bursts in evolution rates.
Venturing into the realm of quantum physics, governed by its own set of physics rules, these researchers wanted to explore the question of whether a quantum system of microscopic components could synchronize into a macroscopic avalanche? So they designed an experiment to investigate domain wall activity in a ferromagnet at very low temperatures, and discovered two types of Barkhausen avalanche events in this quantum regime. In each of these, up to 1016 (10 million billion) electronic spins were flipping in what Philip Stamp realized was a coordinated ‘cooperative quantum tunneling’ process. Just like a conventional avalanche, this process began very small – with the appearance of what Philip called ‘magnetic plaquettes’, ie., small discs of spins which grew off an existing domain wall (see Figures 1a and 1b, below), allowing it to move. To grow, these discs had to overcome an energy barrier – but they did this by ‘quantum tunneling’ through the barrier. Then, long range dipolar forces between the plaquettes allowed them to tunnel cooperatively, and in a process akin to the collapse of a huge array of dominoes, a massive cascade of tunneling events led to a quantum avalanche of moving walls – with the system remaining in the quantum regime throughout.
[Fig. 1a) A single disc shaped “plaquette” where a domain wall has shifted, or has grown away from the original domain wall.]
[Fig. 1b) A pair of domain walls with a pair of "plaquettes" attracting each other.]
Philip remarks, "This is the first example ever seen in nature of such a large-scale cooperative quantum phenomenon, by far the largest macroscopic quantum phenomenon observed in the lab to date."
Tom explains, “we saw this as an opportunity to explore quantum effects bridging the microscopic behavior of a few electron spins and the macroscopic behavior of large domains of spins. Even when there are billions of spins cascading, the voltage signals are very small, so it took us some time to develop the detection ability necessary to accumulate statistically significant data. On the theory side, it required a new approach to magnetic avalanches that had not been formulated previously.”
“To understand how the cooperative tunneling actually works was essentially a piece of deduction”, Philip noted. “A key clue was the existence of 2 kinds of avalanche, with one of them suppressed by a small magnetic field. “From my point of view – the experiments existed and the experimental group couldn’t make sense of them…I realized that one could have either cooperative tunneling or not, and the former was suppressed by the applied field. Working out the details was good fun.”
Tom Rosenbaum adds to the excitement, noting, This “quantum Barkhausen noise, where domain walls [cooperatively] tunnel through barriers rather than being thermally activated over them, has not, to the best of our knowledge, been seen before.” Apart from its intrinsic novelty, there are some surprising features, he declares, including the fact that “one can get quantum nucleation on a large scale, with temperature independence over a wide range of temperature, and with sensitivity to extremely small applied fields.”
Future of Cooperative Macroscopic quantum Phenomena
This discovery not only paves the way for further exploration into cooperative macroscopic quantum phenomena in magnetic systems but also extends to other domains. For Tom, this includes “exploring experimentally the full avalanche spectrum and the long-range interactions that produce collective behavior.” For Philip, there are clear possibilities that similar quantum avalanches may be seen in superfluids and superconductors, and in neutron stars, with scope for many new kinds of cooperative quantum phenomena. But “the most exciting possibility for me is that they will lead us to a new understanding of quantum measurements, and new kinds of quantum measurement – this will allow us to think about quantum mechanics in a new way.”
Stamp concludes, "There is also the interesting prospect that this will suggest new ways of thinking about quantum gravitational phenomena – although such ideas will be very hard to test in experiment! This shows the enormous value of using condensed matter systems as test beds for more exotic phenomena in nature."
Learn More:
- Review the full research article from PNAS, accessible via UBC libraries
- For more information, please access "Quantum Barkhausen Noise Induced by Domain Wall Co-Tunneling: Supplementary Information" (link provided on research article) and Barkausen Noise Dataset (https://doi.org/10.22002/n3n50-msf83)
- Read Philip Stamp's webpage: https://phas.ubc.ca/~stamp/index.html
- Read Tom Rosenbaum's Caltech faculty webpage: https://pma.caltech.edu/people/thomas-f-rosenbaum
Links:
- Read the Physics World article here: https://physicsworld.com/a/quantum-barkhausen-noise-detected-for-the-first-time/
- Interesting Engineering article: https://interestingengineering.com/science/caltech-magnetic-avalanche-quantum-effects
- Science Daily article: https://www.sciencedaily.com/releases/2024/03/240328162625.htm